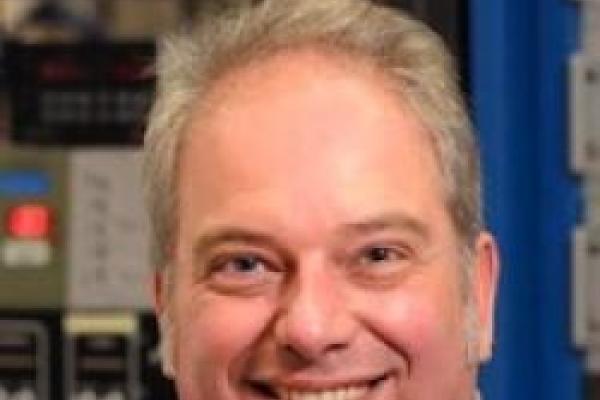
The imminent breakdown of Moore’s law due to heat management bottleneck drives the search for alternative approaches for information encoding and processing. Towards this end, spin waves are very attractive; especially since magnetic insulators, such as oxide-based ferrites and garnets, have very low damping of magnetization dynamics and thus long coherence lengths and times. Nevertheless, in order to integrate devices based on spin waves with already existing electronics it is necessary to manipulate spin waves with charge currents and voltages. This presentation will discuss two possible pathways of electric spin wave manipulation via spin Hall effects and Oersted magnetic fields.
In conductors with strong spin-orbit coupling, spin Hall effects intermix spin and charge currents. Our recent work has focused on characterizing spin Hall effects, which are the key enabling phenomena for insulator based spin-wave devices. In order to gain insight into the underlying physical mechanism and to identify technologically relevant materials, it is important to quantify the spin Hall angle γ, which is a direct measure of the charge-to-spin conversion efficiency [1]. We developed a measurement approach based on spin pumping, which quantifies even small spin Hall angles with high accuracy [2–4]. Furthermore, we show how these spin Hall effects can be used to tailor magnetization dynamics in an adjacent ferrimagnetic insulator, such as yttrium iron garnet (YIG). By using Pt/YIG bilayers, we show how charge currents in the Pt can either reduce or increase the line-width of the ferromagnetic resonance in YIG [5].
Aside from spin accumulations from spin Hall effects, charge currents are also always accompanied by Oersted magnetic fields, which may influence spin wave behavior. This can be used for guiding spin waves through curved waveguides, where otherwise the spin wave propagation is suppressed by the inherently anisotropic spin wave dispersion [6]. This same concept can be further generalized for switching spin waves between two waveguides. Furthermore, I will also demonstrate how thermoelectric effects can be used for detecting spin waves with arbitrarily small wavelengths [7].
This work was supported by the U.S. Department of Energy, Basic Energy Sciences under Contract No. DE-AC02-06CH11357.
[1] A. Hoffmann, IEEE Trans. Magn. 49, 5172 (2013)
[2] O. Mosendz, J. E. Pearson, F. Y. Fradin, G. E. W. Bauer, S. D. Bader, and A. Hoffmann, Phys. Rev. Lett. 104, 046601 (2010).
[3] O. Mosendz, V. Vlaminck, J. E. Pearson, F. Y. Fradin, G. E. W. Bauer, S. D. Bader, and A. Hoffmann, Phys. Rev. B 82, 214403 (2010).
[4] V. Vlaminck, J. E. Pearson, S. D. Bader, and A. Hoffmann, Phys. Rev. B 88, 064414 (2013).
[5] Z. Wang, Y. Sun, Y.-Y. Song, M. Wu, H. Schultheiß, J. E. Pearson, and A. Hoffmann, Appl. Phys. Lett. 99, 162511 (2011)
[6] K. Vogt, H. Schultheiss, S. Jain, J. E. Pearson, A. Hoffmann, S. D. Bader, and B. Hillebrands, Appl. Phys. Lett. 101, 042410 (2012).
[7] H. Schultheiss, J. E. Pearson, S. D. Bader, and A. Hoffmann, Phys. Rev. Lett. 109, 237204 (2012).