The new millennium witnessed the addition of the word "attosecond" (1 as = 10-18 s) to the vocabulary of physics.
Light pulses with attosecond duration are generated either by nonlinear frequency conversion of an ultra-short infrared pump pulse or Fourier synthesis of broad bandwidth radiation, whereas the shortest laser pulses are ~1fs as seen in the time scale below.
Attosecond light pulses are an important scientific advance because the duration corresponds to the electron motion time-scale in the atomic ground state (atomic unit of time = 24 as).
The goal of attosecond science is to observe and control ultra-fast electron motion on the natural atomic timescale.
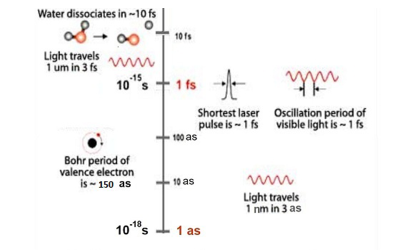
Subtopics in attosecond physics
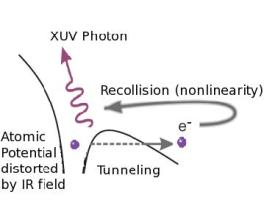
High-order harmonic generation (HHG) up-converts the frequency of a laser, and enables the production of extreme-ultraviolet (XUV) and X-ray light.
It is a hallmark of strong-field and attosecond science, and a promising high energy photon source for laser lithography.
HHG in solids was first observed in the Agostini-DiMauro lab in 2011 (Nat. Phys. 7, 138-141 [2011]), trigging a series of intensive studies that extended our understanding of ultrafast strong physics into the condensed phase matters. In our lab, we drive HHG in solids using long wavelength light sources, including mid-infrared (MIR), long-wavelength infrared (LWIR), and sub-terahertz (THz) radiations.
We have built a novel two-color platform that combines an intense MIR field with a relatively weak sub-THz field, allowing us to study the laser-driven strong field process subjected to a Keldysh extreme low-frequency perturbation.
A long-wavelength optical parametric amplifier (OPA) supplies ~10 µm, ~100 µJ, sub two-cycle LWIR pulses.
The LWIR field is particularly good for the strong-field study of very small bandgap dielectrics or Dirac materials.
Our research focuses include studying the HHG mechanism and the wavelength dependence, exploring the attosecond electron dynamics during the HHG process, probing the intrinsic properties of the medium via HHG spectroscopy, etc.
We closely collaborate with the condensed matter groups to explore non-trivial materials, such as strongly correlated Mott insulator (NiO) and three-dimensional topological insulator (Bi2Se3).
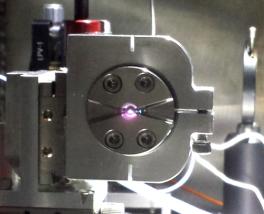
Many experimental and theoretical investigations have been conducted over the past 30 years that have resulted in a comprehensive understanding of a single atom interacting with an intense laser field.
Similar efforts have been invested in the more applicable subject of intense laser-solid interactions but the degree of understanding and control is significantly less due to the complexity associated with this problem.
Nano-scale, Van der Waal bonded atomic clusters generated in high-pressure gas jets provide an interesting alternative target for the study of laser-matter interactions.
Using clusters, one has an experimental local target that is of solid density (n ~ 1022 cc-1) but exists in an environment of low mean background density (n ~ 1014-17 cc-1).
This means that the energy coupling is similar to that in a solid, but without the difficulty of transmitting the light into a bulk target.
In addition, it has been theorized that the conversion of the laser field into high harmonics is greater with clusters than with single atoms (typical efficiency ~ 10-7).
The clusters are considerably smaller than the laser wavelength, contain a significant number of active electrons at effectively the same position in the laser field, and each of these electron oscillators may coherently contribute to a global cluster dipole, potentially increasing HHG efficiency.
This makes clusters of several thousand atoms an exciting nonlinear medium for HHG.
Currently we are pursuing research into the fundamental physics associated with laser-cluster interactions and HHG in clusters.