We have developed the Quantum Trajectory Selector (QTS) to explore and manipulate electron dynamics in quantum processes.
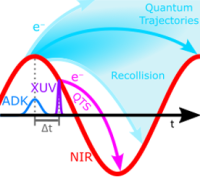
Central to the QTS is synergy of XUV attosecond pulse trains (APT) and strong near-infrared (NIR) fields, enabling us to control/select electron trajectories with attosecond precision during light-matter interactions.
Our setup, featuring a Mach-Zehnder type interferometer with specialized holey mirrors, ensures exact synchronization of NIR and XUV pulses.
The XUV pulses are generated by high harmonic generation and the bandwidth is sculpted by the metal filter and XUV focusing mirror with a multilayer dielectric coating.
At the target, the ionization is initiated by the XUV pulse, with the delay-controlled NIR field then taking the reins to directs the electron trajectories.
A dual time-of-flight system captures the electrons and ions, providing a comprehensive view of the interaction outcomes.
The QTS's innovative design allows for the exploration of complex quantum processes in a way that was once thought to be beyond our reach.
It opens up exciting new possibilities for advancing our understanding of quantum dynamics, particularly in the fields of strong field physics, ultrafast science and quantum simulation.
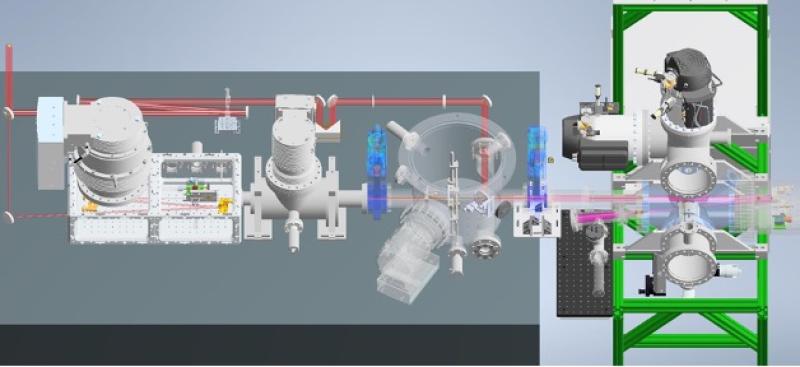
The attobeamline is an experimental apparatus which utilizes the RABBITT (Reconstruction of Attosecond Beating By Two-photon Transitions) technique to measure either the ionization delays of atoms and molecules on the attosecond timescale.
The beamline contains a passively stabilized interferometer (<50 as stability) with single attosecond delay precision which is entirely under high vacuum.
One of the arms is configured with a focusing optic and continuous or pulsed gas jet to perform HHG (high-harmonic generation).
The XUV (extreme ultraviolet) light from HHG is recombined with the fundamental pulse and focused via a 750 mm gold plated toroidal mirror into a 1 m MBES (magnetic bottle electron spectrometer).
The MBES is shielded by mu-metal to remove stray fields and can retard or accelerate the electron kinetic energy over 10s of eV.
The attobeamline can be utilized with light ranging from the ultraviolet (392 nm) to the NIR (2400 nm) with single nm selection over certain regions (1200-2400 nm and 450-600 nm).
These wavelength ranges allow us to finely sample both the ionization delays of molecules studied in the MBES or the RDME (recombination dipole matrix element phase) phase for molecules used as generators in HHG.
For molecules studied with HHG, a technique known as high-harmonic spectroscopy, we additionally have the capability to impulsively align the sample.
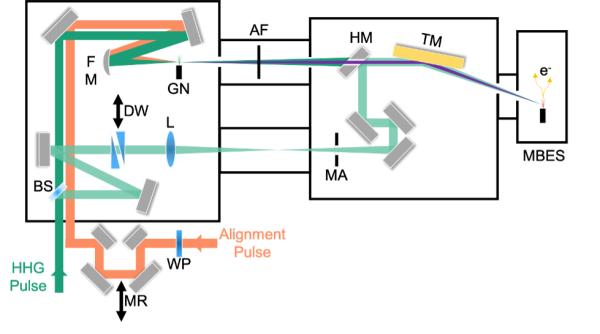
High harmonic generation (HHG) has been a powerful tool in ultrafast physics giving insight into electron dynamics in various media.
The mechanism of HHG has been well understood in gas phase and extensively studied in solids but there’s very little understanding of the HHG process in liquid phase.
In our lab, we’ve built a vacuum apparatus to study HHG in liquid phase.
We use a mid-infrared driving laser to irradiate a sub-micron thin liquid sheet and then collect the generated harmonic spectra on a spectrometer measuring a 10-30 eV photon range.
Studying the mechanism of HHG in liquids could act as a probe of liquid structure and better understanding low-energy electron dynamics in liquid phase.
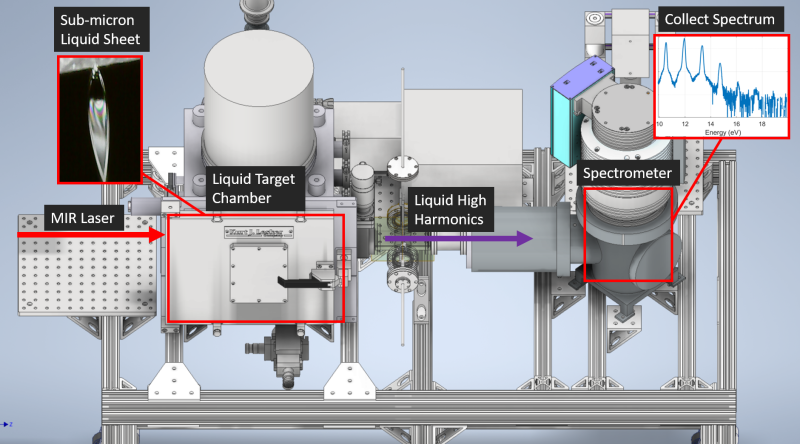
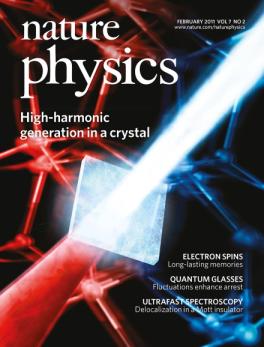
High-order harmonic generation (HHG) up-converts the frequency of a laser, and enables the production of extreme-ultraviolet (XUV) and X-ray light.
It is a hallmark of strong-field and attosecond science, and a promising high energy photon source for laser lithography.
HHG in solids was first observed in the Agostini-DiMauro lab in 2011 (Nat. Phys. 7, 138-141 [2011]), trigging a series of intensive studies that extended our understanding of ultrafast strong physics into the condensed phase matters.
In our lab, we drive HHG in solids using long wavelength light sources, including mid-infrared (MIR), long-wavelength infrared (LWIR), and sub-terahertz (THz) radiations.
We have built a novel two-color platform that combines an intense MIR field with a relatively weak sub-THz field, allowing us to study the laser-driven strong field process subjected to a Keldysh extreme low-frequency perturbation.
A long-wavelength optical parametric amplifier (OPA) supplies ~10 µm, ~100 µJ, sub two-cycle LWIR pulses.
The LWIR field is particularly good for the strong-field study of very small bandgap dielectrics or Dirac materials.
Our research focuses include studying the HHG mechanism and the wavelength dependence, exploring the attosecond electron dynamics during the HHG process, probing the intrinsic properties of the medium via HHG spectroscopy, etc.
We closely collaborate with the condensed matter groups to explore non-trivial materials, such as strongly correlated Mott insulator (NiO) and three-dimensional topological insulator (Bi2Se3).