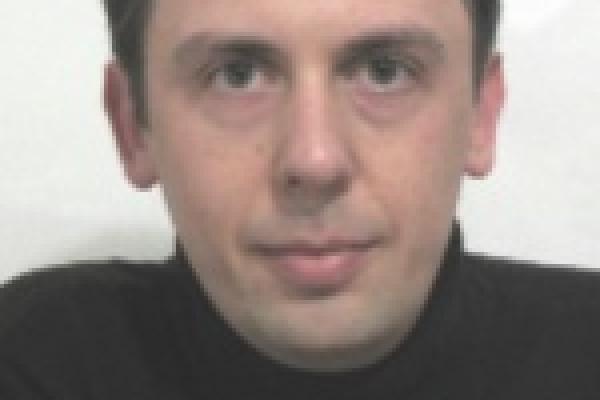
While the term “Spintronics" was originally introduced as label for technologies that represent information through spin states rather than charge states, it is nowadays oftentimes used solely in the context of spin-polarization, spin-injection, and spin-transport effects, for all of which spin-orbit interaction plays an important role. Many organic semiconductors display only weak spin-orbit coupling and charge transport via hopping through localized electronic states which are exposed to hydrogen induced strong local and random hyperfine fields. These materials therefore appear at first glance to be entirely unsuitable for spintronics. However, they also exhibit pronounced spin-related effects not seen in materials with strong spin-orbit coupling1-3 which can be used for alternative, different approaches to spintronics based, for instance, on spin-permutation symmetry states of charge carrier pairs which, in contrast to spin-polarization states, are not directly dependent on temperature and magnetic field strength4. Weak spin-orbit coupling can also promote long spin-coherence times and thus, allow for electrically readable spin memory of electron-5 or nuclear-spins6. The successful implementation of organic spintronics will require a fundamental understanding of the microscopic electronic processes that are to be utilized for such technologies. In this presentation, some of the progress as well as challenges7 for the exploration of these spin-dependent processes will be reviewed. Measurements of spin-coupling types and strengths of charge carriers will be presented2 and examples for unusual physical behaviors of these materials will be discussed, such as an electrically detectable spin-Dicke effect caused by charge carrier spin collectivity8.
[1] D. R. McCamey et al., Nature Materials, 7, 723 (2008); [2] D. R. McCamey, et al., Nature Commun. 6:6688, 7688 (2015). [4] W. J. Baker et al., Nature Commun. 3, 898 (2012); [5] W. J. Baker et al., Phys. Rev. Lett. 108, 267601 (2012); [6] H. Malissa, et al., Science 345 1487, (2014); [7] C. Boehme and J. M. Lupton, Nature Nano. 8, 612 (2013); [8] D. P. Waters, et al., Nature Physics 11, (11) 910 (2015).