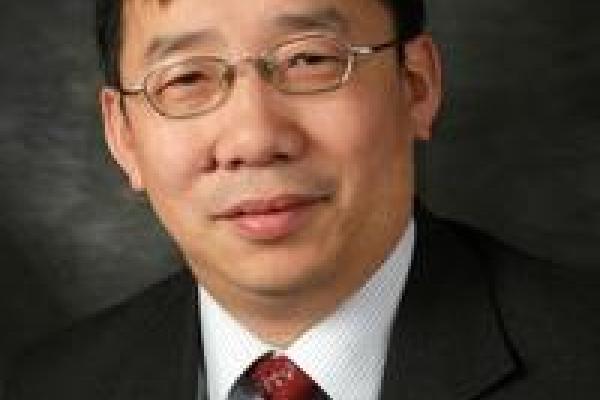
A metallic nanocavity can squeeze the visible light into highly confined intensive plasmon with a broad energy distribution. Such a unique excitation source can lead to many exciting new applications. One of the representative applications is the well-established surface enhanced Raman spectroscopy (SERS) that takes advantage of the high intensity of the plasmon to achieve effective detection of a very small numbers of molecules. I will present here our several new exciting findings from the use of NCP. Our combined theoretical and experimental studies reveal that the NCP acts like a tunable, strong and ultra-fast electromagnetic source that can naturally alter the color of the emission of a molecule[1,2]. The amplified light emission of the molecule enables us to image the coherent dipole-dipole interaction between molecules[3]. Moreover, we found that the NCP can even produce a completely new physical process, namely the nonlinear inelastic electron scattering[4]. We have also developed a new theory that takes into account the locality of the highly confined field to describe the light-matter interaction[4], which has successfully reproduced experimentally observed super high spatial resolution Raman images of a molecule, below one nanometer [5]. It is found that the non-resonant Raman images should be capable of revealing the vibrational modes of the molecule in real space[4b].
References:
1. Z.C. Dong, et al. Nature Photonics, 4 (2010) 50
2. (a) G.J. Tian, et al., Phys. Rev. Lett., 106 (2011) 177401; (b) G.J. Tian, et al., Angew. Chem. Int. Ed., 52 (2013) 4814
3. Y. Zhang, et al., Nature, 531 (2016) 623
4. C.K. Xu, et al. Nature Physics, 10 (2014) 753.
5. (a) S. Duan, et al., J. Am. Chem. Soc., 137(2015) 9515; (b) S. Duan, et al., Angew. Chem. Int. Ed., 128 (2016) 1053.
6. R. Zhang, et al. Nature, 498 (2013) 82; S. Jiang, et al. Nature Nanotechnology, 10, (2015) 865