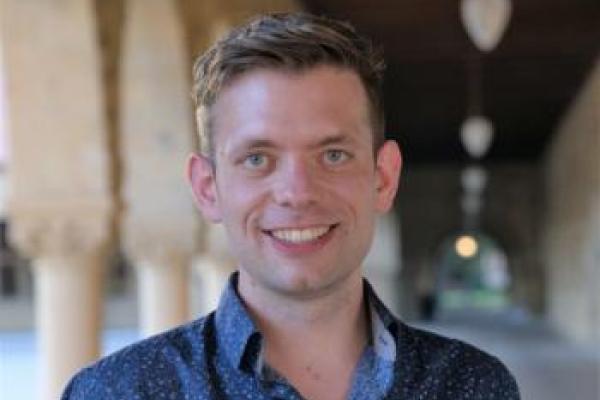
Dr. Christian Heide
Stanford University
Steering electrons with lightwaves: from petahertz electronics to quantum spectroscopy
Location: 1080 Physics Research Building
Faculty Host: Fengyuan Yang
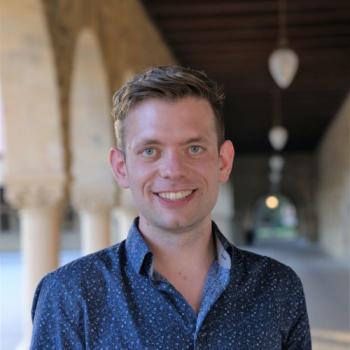
Abstract: In the quest to observe and exploit ever-faster physical phenomena, ultrashort light pulses with a few optical cycles play a crucial role. Precisely controlling the light waveform allows controlling processes inside of solids on a sub-cycle timescale of the laser pulse, giving access to the attosecond timescale. Such waveform control opens prospects for technological applications, especially for signal processing at speeds that exceed by orders of magnitude the current gigahertz clock rates of processors, opening the realm of petahertz electronics. In my talk, I will present how the electric field of strong laser pulses can be used to build ultrafast light-wave electronics and how we can use the underlying electron dynamic to observe and control emerging quantum processes in various material systems.
Using a strong light field, typically in the order of 1 V/nm, it is possible to steer electrons on complex electron trajectories inside solids, such as in graphene [1-3]. Due to the fast oscillating nature of the lightwave, the electron dynamics can be described as fully quantum mechanical, which allows the electron to drive like a matter wave through the band structure. In the vicinity of the band gap, the electron wavefunction can be split into conduction and valence band states. Because these transition events act as electron beam splitters, we have built a light-field-driven electron interferometer akin to a Mach-Zehnder interferometer for light. The quantum phase accumulated in the beam-split state determines in which output port the electron ends up, in the valence or the conduction band, and defines the direction and amount of injected photocurrent [1, 2]. Additionally, by tailoring the electron trajectory with the light field, we can steer the electrons through the entire Brillouin zone and precisely map the accumulated quantum phase, offering a novel coherent spectroscopy platform.
Although the band structure of graphene allows quantum control most simply, it is even more fascinating to extend the concept of light-field controlled electron dynamics to quantum materials and topologically protected materials. These materials are promising candidates for Floquet engineering or non-dissipative topological quantum electronics [4, 5]. Based on bismuth selenide, I demonstrate that the topologically protected conducting surface states are ideally suited to coherently drive electrons inside of solids [5]. Additionally, I demonstrate that light-field-driven electron dynamics are ideally suited to measure the coherence lifetime of the electrons after excitation [6, 7], to build a novel quantum sensor for the electro-optical reconstruction of the band structure, to control the charge on the attosecond timescale across heterostructures [8] and to built light-field driven logic gates [2].
Looking forward, I will discuss how to bridge the gap between ultrafast phenomena, photonics, and quantum materials towards LightwaveControl: Lightwave Quantum Spectroscopy and Electronics. The primary goal of my research will be to harness strong light fields to dynamically control and imprint information on electrons on the fastest possible timescale. This includes strategies to read out the quantum phase by developing advanced ultrafast spectroscopy tools such as light-field-driven electron interferometry and petahertz field sampling. Access to the quantum phase and understanding lightwave-driven processes in tailored quantum materials will allow new insights into material properties, which will ultimately help to develop electronics operating at optical clock rates.
[1] Higuchi T., Heide C., et. al. Nature 550, 224-228 (2017) [2] Boolakee T., Heide C., et al. , Nature 605, 251-255 (2022)
[3] Heide C., et. al PRL 121, 207401 (2018) [4] Kobayashi Y, Heide C., et al. Nature Physics 19, 171-176 (2023)
[5] Heide C., Kobayashi Y, et al. Nature Photonics 16, 620-624 (2022) [6] Heide C., Eckstein T., et. al., Nano Letters, 21, 9403 (2021)
[7] Heide C., Kobayashi Y, et. al. Optica 9, 512-516 (2022) [8] Heide C. et. al. Nature Photonics 14, 219–222 (2020)
Bio: Christian Heide received his PhD from Friedrich-Alexaner University in Erlangen- Nuremberg, Germany, in 2020 with departmental distinction. Since 2020, he has been working with Profs. Tony Heinz, David Reis, and Shambhu Ghimire at the Stanford PULSE Institute. Since 2021, he has been a Feodor Lynen Fellow of the Alexander von Humboldt Foundation. Christian's research interests include coherent control of electrons on the fastest possible timescale. This comprises the design and development of light-field-driven electronics and the development of advanced spectroscopy tools to probe novel quantum phenomena. Christian was awarded the2021 prize for the best dissertation in the field of solid-state physics by the German Physical Society (DPG) and the German Society for Applied Optics.