Physicists tend to figure out how a system works by breaking it into smaller components. Usually, the apparent complexity of a large system can be understood in terms of simpler interactions between these more fundamental building blocks. Think of the amazingly complex and varied interactions between protein molecules, which arise from much simpler interactions of just a few types of atoms.
Indeed, each atom itself exhibits highly nontrivial properties, which can be studied and understood in terms of its fundamental components: the electrons and the nucleus. By separating these elements, we find a fundamental force at play – Coulomb’s Law of attraction and repulsion – that combines with quantum mechanics to explain the atom’s properties.
We can delve further...The atomic nucleus itself is hugely complex, but can be understood in terms of only two components – the protons and the neutrons – and the forces between them. We can understand a lot by systematically taking the nucleus apart.
“Our group uses the world’s largest machines to probe the Universe’s smallest systems and most intense forces through ultrarelativistic, violent collisions of atomic nuclei. The breadth of our expertise — in detector construction, experimental operations, data analysis and phenomenology — allows us to play leading roles in several experiments.”
-Mike Lisa
College of Arts and Sciences Distinguished Professor, Department of Physics
But we’re not done yet. It turns out that protons and neutrons are complex, and part of a much larger and fascinating subatomic “zoo” of particles which are – you guessed it – composed of what we believe are truly fundamental particles, quarks and gluons. However, here the physicist’s game of separating the constituents to study them individually ends. Due to a fascinating feature of the Strong Force, a quark cannot exist in isolation. If we try to remove a quark from a proton, new quarks pop into existence from the vacuum (and Einstein’s famous E = mc2) and immediately recombine with the original.
The Strong Force is the most complicated and – well – the strongest of Nature’s fundamental forces. It is the special properties of the Strong Force – not the Higgs boson! – that give rise to more than 90% of the mass in the visible universe. It is also by far the least-understood force in Nature.
Professors Daniel Brandenburg, Tom Humanic and Mike Lisa lead Ohio State’s experimental high energy nuclear physics (HENP) group. Supported by grants from the Department of Energy, they perform experiments at the Relativistic Heavy Ion Collider (RHIC) at Brookhaven National Laboratory in New York and the Large Hadron Collider (LHC) at CERN in Europe. The experiments themselves are huge, resembling particle physics experiments in size and complexity. However, the focus is quite different; rather than discovering new particles, the HENP group probes unique properties of the Strong Force itself.
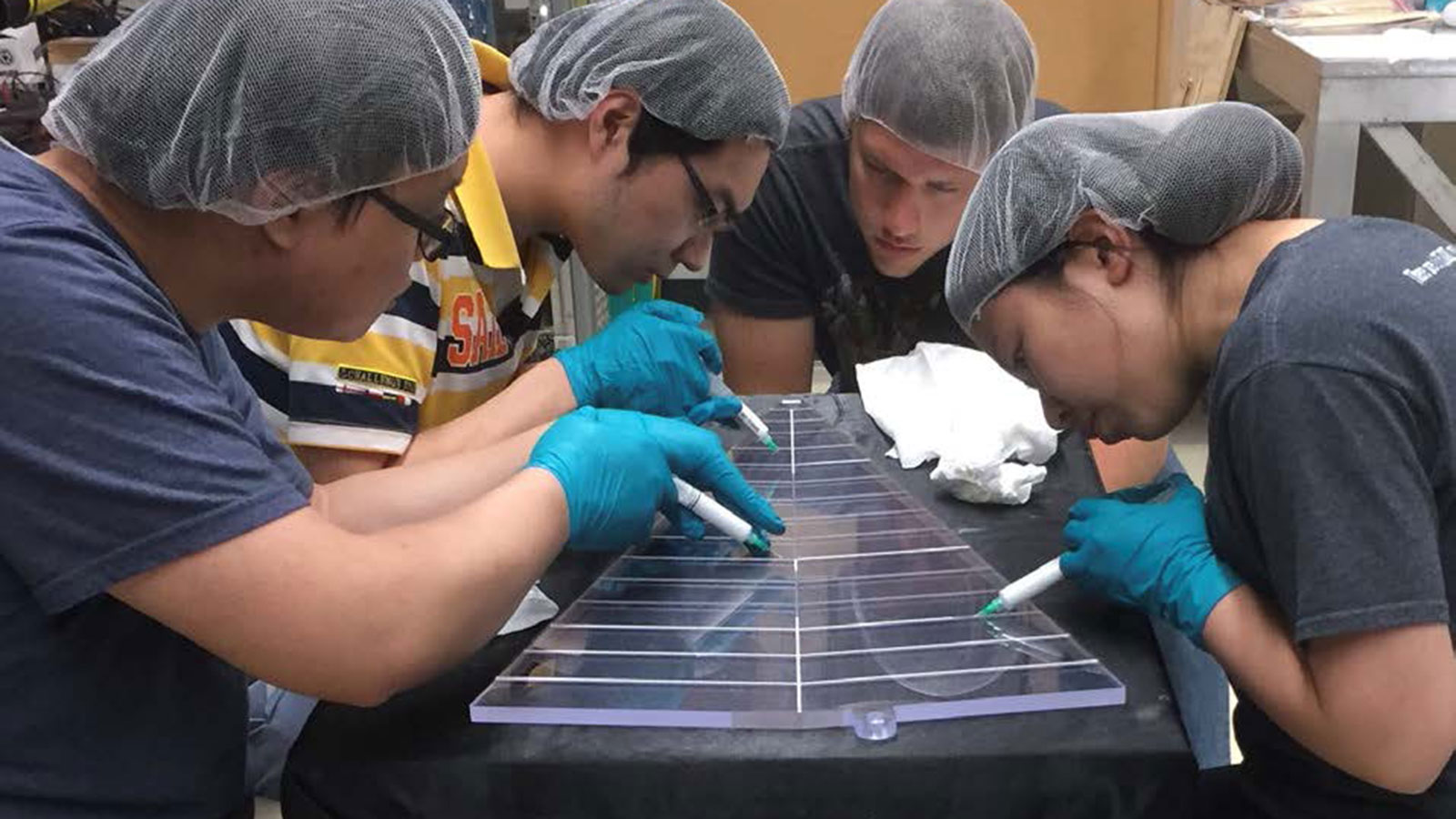
Students building the STAR Event Plane Detector. Ohio State is the lead institution on this detector: constructing, maintaining and operating it during RHIC beamtime. The EPD is crucial to measurements that connect the initial state geometry of the collision to the reaction of the quark-gluon plasma.
These experiments are huge in terms of the number of people involved, too. The HENP group actively collaborates with hundreds of physicists around the world and are prominent leaders in the community, with members regularly holding leadership positions such as physics analysis coordinator, physics working group convener and project leader for experimental systems. The group’s reputation gives Ohio State students increased visibility and opportunities; young HENP members regularly travel to international workshops to present their new results. Students learn how to effectively work in large, distributed scientific teams.
Students work on a wide array of analyses, including femtoscopy, the use of quantum correlations to measure spacetime features of subatomic collisions; momentum flow; searches for novel quark-gluonic states; novel physics in ultraperipheral collisions; and polarization measurements. These require extensive computational and analytic skills, as well as intimate knowledge of detector operation.
Members of the group have made several high-profile discoveries in the past few years. Humanic’s team published the first compelling evidence of tetraquark formation in collisions at the LHC. Lisa’s team published the discovery of “vorticity” (rotational flow substructure) in the quark-gluon plasma, which is – amazingly – a fluid 10,000 times smaller than a single atom! Brandenburg uncovered evidence of light-on-light scattering and vacuum birefringence in the interaction between intense electromagnetic fields of nuclei traveling at 99.995% the speed of light. In addition to experimental measurements, group members regularly perform and publish theoretical studies of these phenomena.
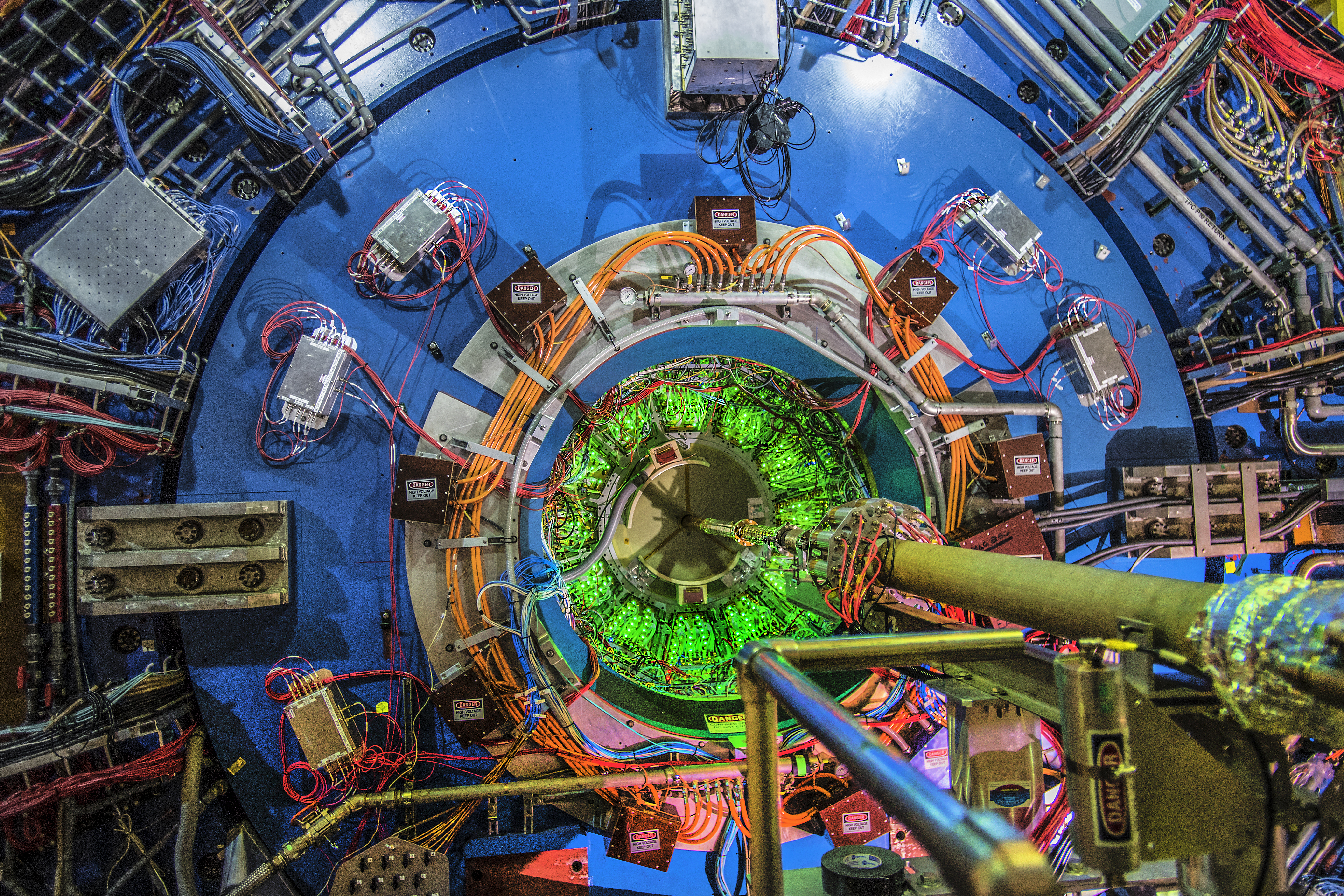
Nuclei traveling at 99.955% the speed of light travel down the vacuum tube entering the STAR experiment. They collide at the center of the massive detector, producing thousands of particles that physicists analyze offline.Photo courtesy of: Brookhaven National Laboratory, CC BY-NC-ND 2.0, flickr.com/photos/brookhavenlab
While continuing with RHIC and LHC physics, the group is actively involved in preparations for experiments at the next large nuclear facility, the Electron-Ion Collider (EIC) at Brookhaven Lab. The group is leading the project for a hadronic calorimeter. Students will be heavily involved in detector construction and testing in the lab, as well as software infrastructure for the project. The broad variety of projects in the HENP group provide students with strong computing, analysis and hardware skills that will serve them well in science or industry.