Clocking the movement of electrons inside an atom
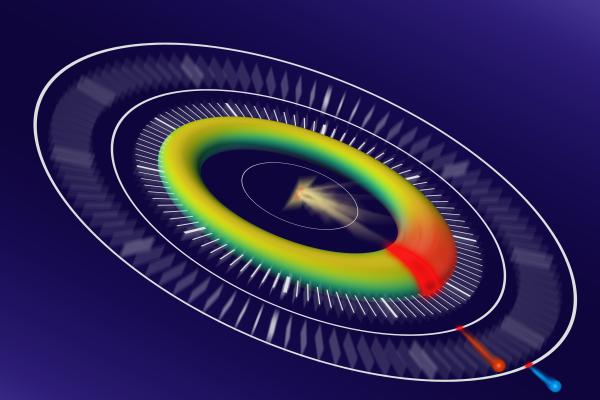
Scientists dramatically enhance the achievable resolution at x-ray free-electron lasers with a new technique
Hard X-ray free-electron lasers (XFELs) have delivered intense, femtosecond X-ray pulses for over a decade. More recently, even attosecond XFEL pulses have become available, cementing these facilities’ importance for ultrafast science.
One of the most promising applications of XFELs is in biology, where researchers can capture images down to the atomic scale even before the radiation damage destroys the sample. In physics and chemistry, these X-rays can also shed light on the fastest processes occurring in nature with a shutter speed lasting only one femtosecond – equivalent to a millionth of a billionth of a second.
However, on these miniscule timescales, it is extremely difficult to synchronize the X-ray pulse that sparks a reaction in the sample on the one hand and the laser pulse which ‘observes' it on the other. This problem is called timing jitter, and it is a major hurdle in ongoing efforts to perform time-resolved experiments at XFELs with ever-shorter time resolution. Now, a large international research team involving collaborators from Ohio State University, DESY in Hamburg, PSI in Switzerland and many other institutions spread across seven countries has found a method to get around this problem at XFELs and demonstrated its efficacy by measuring a fundamental fast decay process in an atom. The work has just been published in Nature Physics. The experiments were conducted using the Linear Coherent Light Source (LCLS) XFEL at SLAC in Menlo Park, CA. The OSU team included Prof. Louis DiMauro, Dr. Cosmin Blaga and former graduate student, Yu Hang Lai.
Many biological systems – and some non-biological ones – suffer damage when they are excited by X-rays. One of the causes of damage is the process known as Auger decay. The X-ray pulse catapults core-level electrons in the sample out of place, leading to their replacement by electrons in outer shells. As these outer electrons relax, they release energy which can induce the emission of another electron, known as an Auger electron. Radiation damage is caused by both the X-rays and the continued emission of Auger electrons, which can rapidly degrade the sample. Timing this decay would help to evade radiation damage.
Ordinarily, timing jitter would appear to preclude time-resolved studies of such a short process at an XFEL. To circumvent the jitter problem, the research team came up with a pioneering, highly precise approach and used it to chart Auger decay. The technique, dubbed self-referenced attosecond streaking, is based on mapping the electrons in thousands of images and deducing when they were emitted based.
For the first application of this method, the team used neon gas, where the decay timings have been inferred in the past. After exposing both types of emitted electrons to an external ‘streaking’ laser pulse, the researchers determined their final kinetic energy in each of tens of thousands of individual measurements. Crucially, in each measurement, the Auger electrons always interact with the streaking laser pulse slightly later than the photoelectrons. This constant factor forms the foundation of the technique. By combining so many individual observations, the team was able to construct a detailed map of the physical process, and thereby determine the characteristic time delay between the photo- and Auger emission.
The researchers are hopeful that self-referenced streaking will have a broader impact in the field of ultrafast science. Essentially, the technique enables traditional attosecond streaking spectroscopy, previously restricted to tabletop sources, to be extended to XFELs worldwide as they approach the attosecond frontier. In this way, self-referenced streaking may facilitate a new class of experiments benefitting from the flexibility and extreme intensity of XFELs without compromising on time resolution.
Image: Artistic depiction of the experiment. The inherent delay between the emission of the two types of electron leads to a characteristic ellipse in the analysed data. In principle, the position of individual data points around the ellipse can be read like the hands of a clock to reveal the precise timing of the dynamical processes.